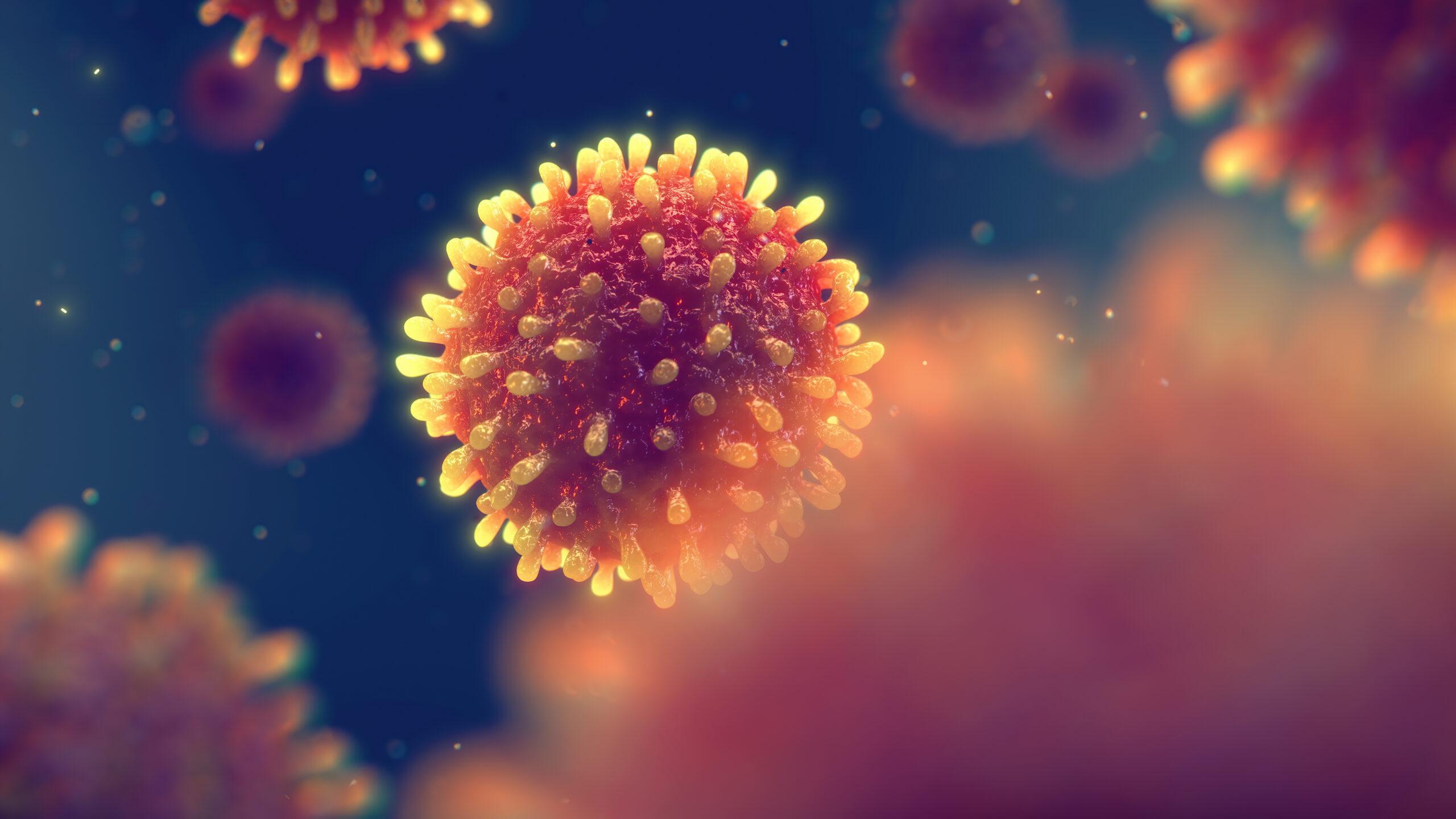
Adobe Stock 508244320
“Tucking In” of Viral Capsid Protein Allows Passage of Giant Structure through Nuclear Pore, Allowing Virus to Take Over
The hepatitis B virus causes about 800,000 deaths per year, on par with HIV and the malaria parasite. However, as it spreads, it performs a trick that may make it vulnerable to new therapies. Instead of importing its proteins and genes separately into the host cell nucleus, it enters more or less whole through the nuclear pore. Using the second-generation Anton supercomputer developed by D. E. Shaw Research and hosted at PSC, teams led by the universities of Alabama and Delaware revealed the likely motion of a viral protein that helps shrink HBV for insertion into the nucleus, identifying a target for future therapies.
WHY IT’S IMPORTANT
Infection by HBV poses a similar threat to human life and health as AIDS and malaria. About 300 million people worldwide are at risk from this virus. It causes 800,000 deaths each year, through severe hepatitis and liver cancer. There is an effective vaccine, but most people don’t receive it and for many others, its protection fades over time.
Most viruses, in entering a host cell, hijack it by inserting their genes and proteins separately into the cell nucleus. There, the viral genes force the cell’s genetic machinery to produce RNA that in turn directs viral protein production. HBV is different. Instead of just entering the nucleus piecemeal, it imports its entire capsid — the protein-covered capsule that contains its genes and proteins — into the nucleus at once, through the nuclear pore. It’s kind of like putting an entire house on a flatbed and driving it to a new property instead of building the house there. Doable, but it stretches the limits.
“What’s unique about this virus is that it enters the nucleus as a whole capsid, a whole particle. Most viruses would uncoat in the cytoplasm, and then all the proteins, one by one, [would] be transported to the nucleus … I think understanding [this] basic science is really vital to devise strategies to prevent [infection by HBV].”
— Gino Cingolani, University of Alabama at Birmingham
Gino Cingolani, a professor of biochemistry and the director of the Center for Integrative Structural Biology at the University of Alabama at Birmingham, had good structural information about most of the capsid — except for one troublesome strand of amino acids called the carboxyl-terminal domain (CTD) of the HBV coat protein Cp183. That’s because the CTD doesn’t stay still for scientists to take its picture with X-rays or other methods. Like a restless toddler, it keeps moving, and winds up so blurry that it’s effectively invisible.
To unravel the mystery of how the giant HBV capsid fits through the nuclear pore, Cingolani joined forces with colleague Jodi A. Hadden-Perilla, an assistant professor and the C. Eugene Bennett Early Career Chair of Chemistry at the University of Delaware, as well as colleagues at Thomas Jefferson University and Indiana University. Together, their teams employed Anton, a special-purpose supercomputer for molecular dynamics simulation that was designed and constructed by D. E. Shaw Research (DESRES). At the time, a second-generation Anton machine (now replaced by an upgraded third-generation Anton) was made available to scientists without cost by DESRES, and was hosted at PSC with operational funding support by the National Institutes of Health.
HOW PSC HELPED
The CTD and its slippery ways posed a difficult computational problem. Its many motions meant that the shape it had to take to allow the capsid through the pore might be fleeting. Simulations by traditional supercomputers, which would take far too much real time to simulate anything more than a few microseconds or less, would likely miss the critical flop that produced the import signal.
Anton at PSC, on the other hand, specializes in long, complex molecular simulations. The second-generation Anton at PSC was capable of simulating large numbers of atoms roughly 10 times faster than general-purpose systems. This gave it the ability to simulate molecules for, in the case of the HBV work, about 30 microseconds in a reasonable amount of real time, revealing rare but pivotal events for a biomolecule’s function.
“On a traditional [supercomputer], we can run simulations of these intrinsically disordered proteins, but these simulations are much shorter than we can obtain on Anton … So what Anton really does for us is help us overcome this barrier to some extent, where we can really start to observe longer timescale behaviors, and we can have more confidence in conclusions we draw, because we have a more comprehensive view of what the protein is doing.”
— Jodi Hadden-Perilla, University of Delaware
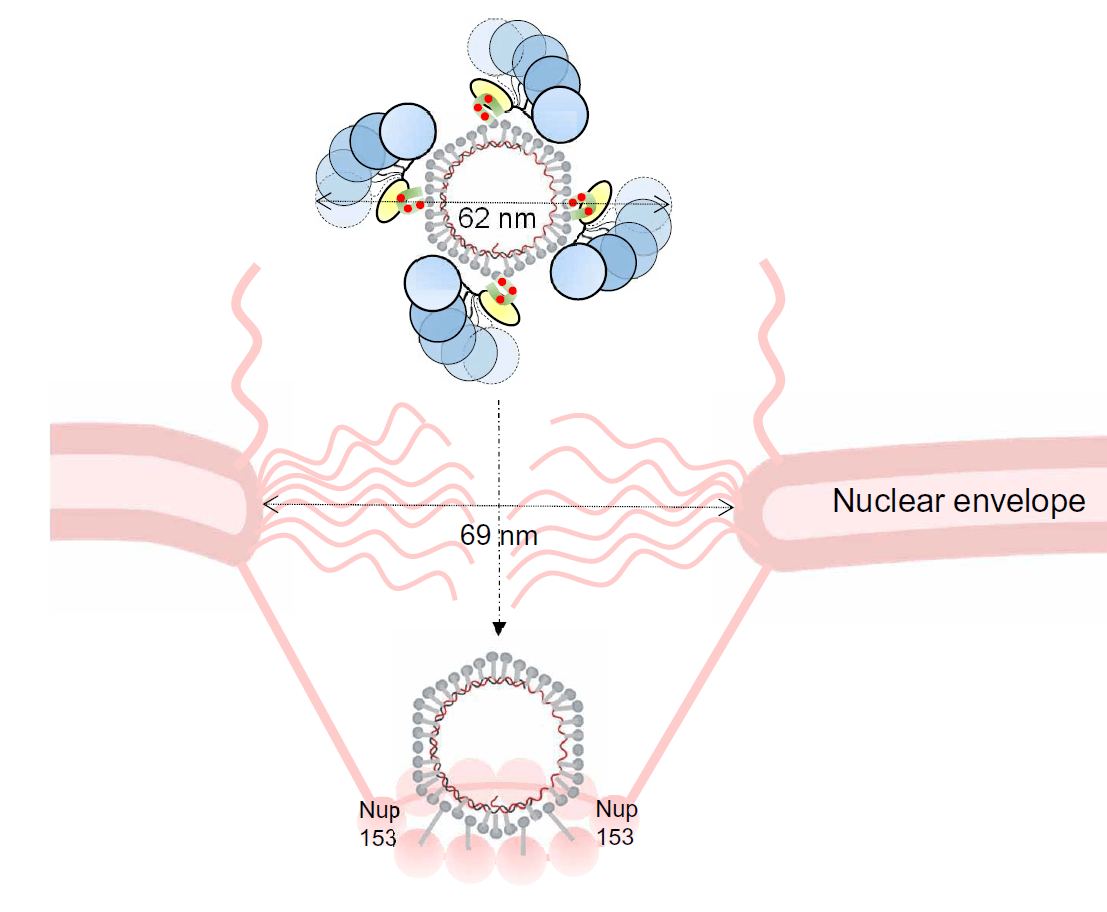
Hadden-Perilla’s Anton simulations helped explain how the capsid manages to slip through the nuclear pore. Scientists had known that, in the cell, the CTD was chemically modified with negative electrically charged phosphate groups. Cingolani had guessed that these groups might pull it toward positively charged amino acids in the CTD, making the protein contract like a diver tucking in for a cannonball. The change would also allow the CTD to stick to the cell’s importin alpha-1 protein, which marks structures for entry through the nuclear pore.
The Anton simulations showed exactly this kind of “tuck.” Together, the contracted capsid and the importin were about 620 angstroms across in size — roughly one 400,000th of an inch. While tiny by normal-life standards, this is close to the size limit for passing through the nuclear pore.
The work offered the first explanation both of how the CTD behaves to trigger import into the nucleus and how the capsid pulls importin in close so together they are just small enough to fit. Once the third-generation Anton arrives at PSC, the team hopes to be able to use it to simulate the entire system in one go, with the second-gen simulations guiding how the newly discovered motions of the CTD should be included. The scientists hope that by understanding the importation of the capsid into the host cell nucleus, doctors can better prevent it and so block a critical step in the virus infecting new cells to persist in a person’s body.