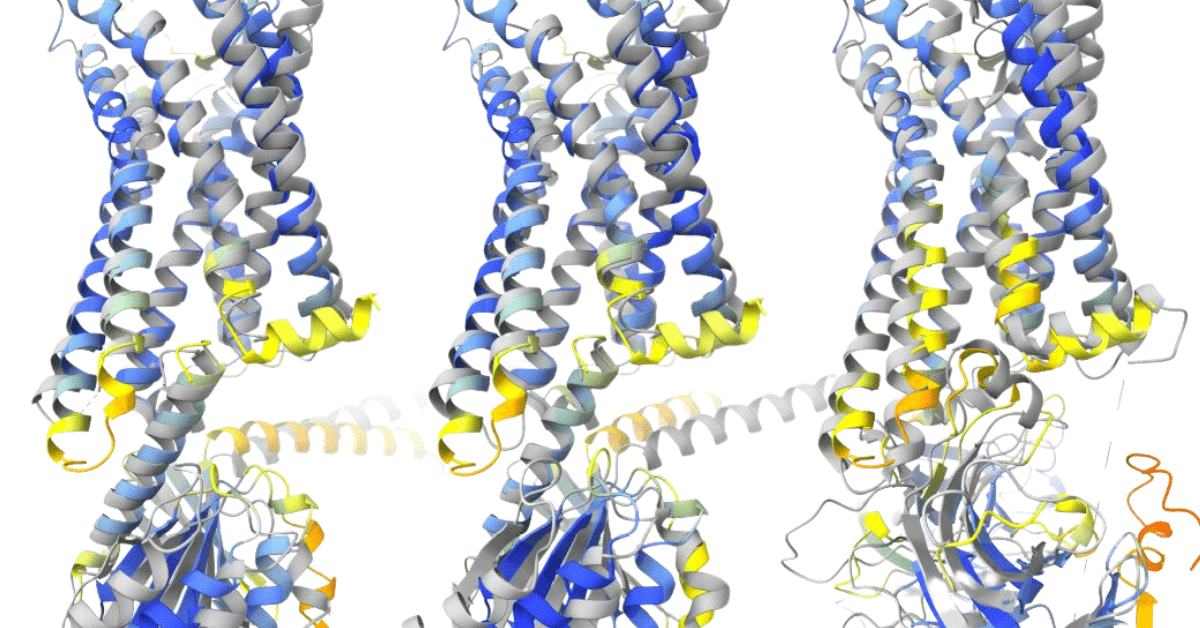
Generated models of the AT1 receptors taken from Supporting Information: Structural Rearrangement of the AT1 Receptor Modulated by Membrane Thickness and Tension, Figure S11.
G Protein-Coupled Receptor Triggered by Mechanical Tension as Well as Chemical Signals, Helping to Regulate Blood Pressure
The G protein-coupled receptor (GPCR) proteins are central to cells’ responses to the body’s messages. But scientists were puzzled at how one particular GPCR in blood vessels responds to mechanical force in addition to chemical signals. A team from Oregon State University (OSU) used a second-generation Anton supercomputer, developed by D. E. Shaw Research and hosted at PSC, to explore how tension affects this protein, uncovering new facets of how GPCRs respond to different signals.
WHY IT’S IMPORTANT
By some estimates, around 700 pharmaceutical therapies work by affecting the function of G protein-coupled receptors — over one-third of all approved drugs. Sitting in the outer membrane of the cell, GPCRs help relay signals from the rest of the body to the inside of the cell. GPCRs affect functions as different as vision, smell, mood regulation in the brain, the body’s immune response to pathogens, signal transmission between nerves, response to hormones, the growth of tumors, and regulation of blood pressure and heart activity.
It’s in that last role that GPCRs showed some odd behavior.
In the smooth muscle cells that line the arteries, a particular GPCR called the angiotensin II type 1 receptor (AT1R) responds to incoming chemical signals from the body via two major pathways. When the messenger molecule angiotensin II sticks to AT1R on the outside surface of the membrane, AT1R activates messengers inside the cell called G proteins, as well as a set of signals known as the beta-arrestin pathway. The two pathways make the muscle cell change its behavior in different ways, controlling the degree to which the muscle cells contract and limit blood flow through the artery.
“Angiotensin II binds to [AT1R, and] will activate it … The tricky part, the part that we’ve become very interested in … is that [AT1R] can also be activated by mechanical stimuli … And this was a bit puzzling, because it sort of went against a lot of what people knew.”
— Juan Vanegas, OSU
The surprise came about 15 years ago, when scientists discovered that AT1R could be made to generate a signal when there was no angiotensin II around to trigger the protein. All you had to do was put the receptor protein and the membrane housing it under tension.
To some extent, the idea that muscle cells that control blood pressure might react to physical force made sense. But researchers were mystified as to how this happened. Also, scientists soon discovered that tension only triggered the beta-arrestin part of its signal, which was also surprising.
Graduate student Bharat Poudel, working in the OSU laboratory of Associate Professor of Biochemistry and Biophysics Juan Vanegas, wanted to find out how tension alone could trigger AT1R. To explore this question, they turned to Anton, a special-purpose supercomputer for molecular dynamics simulation that was designed and constructed by D. E. Shaw Research (DESRES). A second-generation Anton machine is made available without cost by DESRES, and is hosted at PSC with operational funding support by the National Institutes of Health.
HOW PSC HELPED
Creating a simulated AT1R and the membrane containing it in the computer would be complicated, involving lots of factors such as how tension affected the membrane, how the force impacted the protein directly, and how the protein and membrane affected each other. Worse, the molecular movements that govern AT1R’s interaction with its signaling pathways are, by biochemical standards, really slow. The simulations would need to reproduce the molecular motions for tens of microseconds, many times over, to get valid results. This would have taken weeks to months of real time using a conventional supercomputer.
The second-generation Anton machine at PSC can simulate large numbers of atoms roughly 10 times faster than general-purpose systems. This gives it the ability to simulate molecules for microseconds in a reasonable amount of real time.
As a first step, the team simply changed the thickness of the membrane. In the simulations, a thicker membrane helped AT1R change between its active and inactive states more easily. This enabled the protein to transition from the active to the inactive state. With a thinner membrane, the transition was harder for the protein to accomplish in either direction. This tended to lock the protein in an active or inactive state depending on the initial conditions.
“The time scales of all these processes are very, very slow, right? … Running the simulations with GPUs, which will give you the best possible performance in conventional computing, would take us easily multiple weeks to months to be able to run some of the simulations that we would need. [And] you have to run multiple long simulations to really get a picture of what’s happening … It’s probably only a few weeks … to run everything that we need on Anton.”
— Juan Vanegas, OSU
Next, the investigators simulated putting tension on AT1R and the membrane. This forced the membrane to become thinner, but also switched the protein into its active state. Finally, in a separate set of simulations, the team used the artificial-intelligence-based online tool AlphaFold 2 to investigate how binding to G proteins and beta-arrestin affected AT1R’s shape. These results mirrored what was happening in the Anton simulations, giving the scientists more confidence their findings were accurate. The team reported their results in the Journal of Physical Chemistry B in September 2024.
Future studies planned by the team include characterizing the effects of tension and the chemistry of the cell membrane, which can vary in different environments and states of health. Tests on the real AT1R protein will be needed to confirm the results as well. The current simulations also hinted at important motions of the protein that the increased timescales enabled by the third-generation Anton now being put into operation at PSC will reveal. The OSU investigators plan to explore these phenomena more fully.