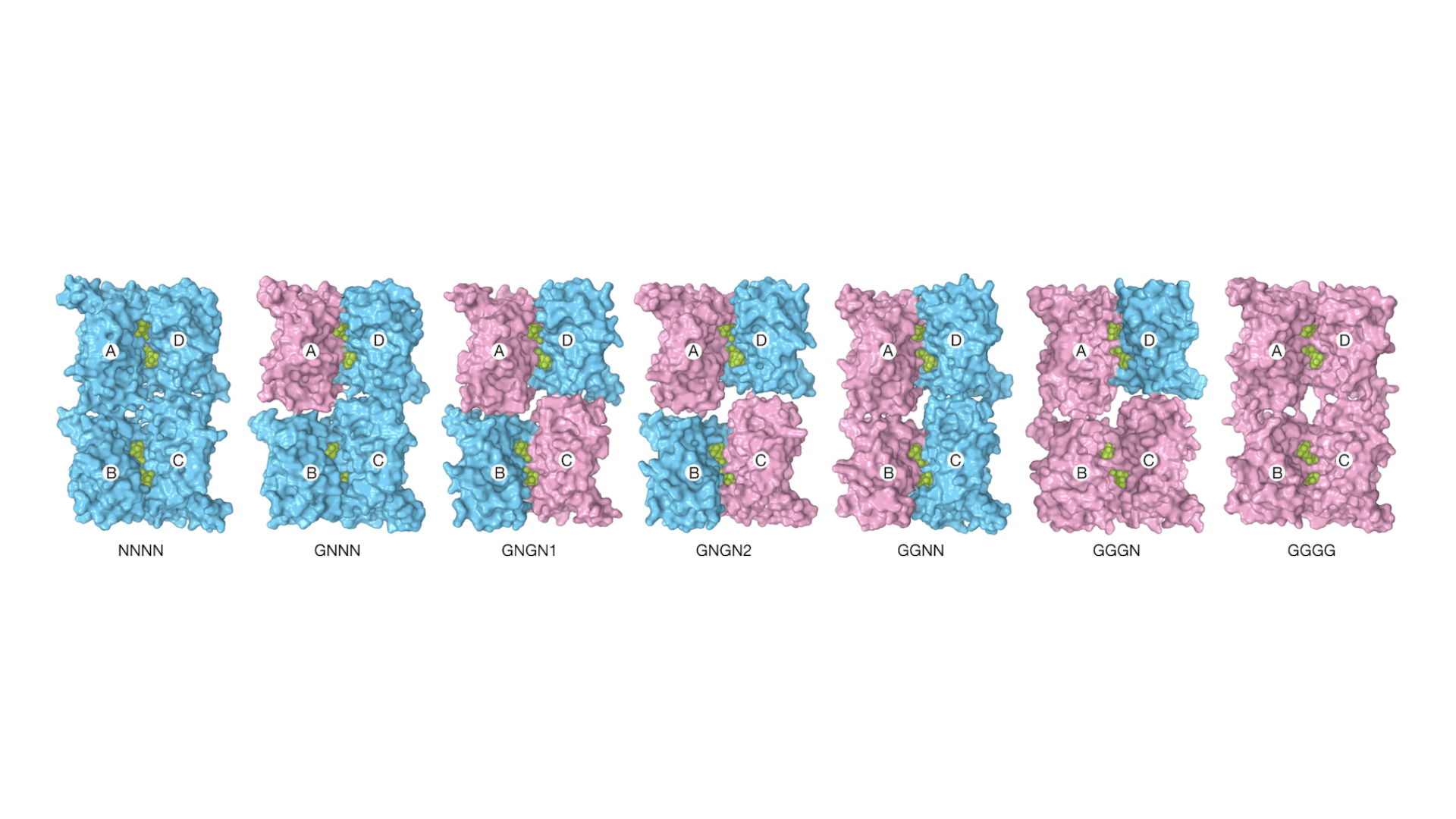
Different numbers of kinks in the iGlu-R protein from glutamate binding to the outside of channel cause its central pore (seen from the top looking down) to open progressively, allowing different levels of ion current to pass through. From Yelshanskaya, M.V., Patel, D.S., Kottke, C.M. et al. Opening of glutamate receptor channel to subconductance levels. Nature 605, 172–178 (2022).
Complementary strengths of researchers, computers help identify structure and motions of protein and its electrical conductance through the nerve-cell membrane
Scientists do not fully understand the iGluR protein in the brain, which underlies some serious forms of epilepsy. To uncover the workings of this protein complex and help design better drugs to treat its dysfunctions, a team of computational chemists from Carnegie Mellon University (CMU) employed simulations on an Anton 2 supercomputer developed by D. E. Shaw Research and hosted at PSC, as well as on the PSC’s flagship Bridges-2 system. Together, these simulations revealed a step-by-step activation of iGluR that can help identify future drug targets.
WHY IT’S IMPORTANT
Every thought, memory, and emotion we have depends on electrical signals shuttling around the brain. Underpinning many of those electrical signals is the ionotropic glutamate receptor (iGluR), a protein that sits in the outer membrane of some nerve cells. Problems with this protein cause epileptic seizures and degeneration of nerve cells.
When an incoming signal arrives from a neighboring nerve cell, the transmitting cell sends glutamate molecules across the narrow gap between the cells, called the synapse. The glutamate then sticks to the receiving cell’s iGluRs. This causes the barrel-shaped protein to open its central channel, letting electrically charged potassium and sodium ions through. The incredibly tiny electrical current of ions through the membranes triggers other, nearby receptor proteins. These in turn cause a wave of electrical activity that sweeps through the nerve cell, eventually causing it to signal other nerve cells downstream.
“When the glutamate receptor is a little bit too excitable, when it stays open too long, it immediately goes to disorders of the brain. There’s one single FDA-approved drug to inhibit [these] receptors, perampanel. But no one knew how it worked on the channel. Sasha [Alexander Sobolevsky] found the site.”
—Maria Kurnikova, CMU
There’s only one drug approved for problems with iGluR, perampanel. While it helps many people with seizure disorders, it can sometimes cause addiction. Doctors would like to have more choices for treating iGluR-based seizures. For that, we need to better understand iGluR.
Working with electrophysiologist and iGluR structure pioneer Alexander Sobolevsky at Columbia University, Maria Kurnikova of the Mellon College of Science at CMU and her graduate students used a combination of the D. E. Shaw Research (DESRES) Anton 2 supercomputer hosted at PSC, PSC’s Bridges-2 advanced research computer, and their own computing “cluster” to thoroughly dissect the motions of iGluR when glutamate attaches to it.
HOW PSC HELPED
The iGluR protein consists of four identical subunits that form the walls of the channel. Sobolevsky’s previous iGluR work had uncovered important clues as to how its four subunits coordinated to carry out the protein’s function. Using cryoelectron microscopy, the Columbia group had produced static pictures of how the amino acid chains of each subunit folded, coming together to form a channel through the cell membrane.
Each subunit of iGluR has a binding site for glutamate on the outside of the cell. Lab experiments had shown that the channel’s gate wasn’t a simple open-or-closed door. The protein lets ions through in four levels, each with increasingly strong current. Scientists suspected that a glutamate sticking to each binding site increased the current by a little bit. But they weren’t sure.
Sobolevsky’s earlier work with the protein showed that, though the four protein subunits in iGluR are chemically identical, they weren’t folding in the same way. Two of them partly stuck out straight from the cell surface. The other two kinked to the side. The kinks had something to do with opening the gate. But in computer simulations, neither the folding of the protein nor the current going through it changed much whether one, two, three, or four glutamates were bound.
Sobolevsky suspected that the structures and the earlier simulations were missing something. He contacted Kurnikova, whose team has deep experience with molecular dynamics (MD) simulations.
“We produced an enormous amount of simulation data using Anton and [Bridges-2] … We needed to see if the channel relaxed into these different gated structures in a correlated manner … It was a difficult problem to simulate the [current] fluctuations, [a] small signal in really noisy data.”
—Maria Kurnikova, CMU
The MD simulations would be some of the most complex that Kurnikova’s team had attempted. Capturing the full movement of iGluR would require very long simulated times. That’s a particular strength of Anton, a special-purpose supercomputer for MD simulation that was designed and constructed by DESRES. That company has made a second-generation Anton 2 machine available without cost, and is hosted at PSC with operational funding support by the National Institutes of Health. The scientists also made use of repeated computations on the National Science Foundation-funded Bridges-2’s powerful graphics processing units to capture the current of potassium and sodium ions through the channel.
In the simulations, all four protein chains of the glutamate-free iGluR were straight. The scientists found a mixture of kinks with various numbers of glutamate bound. An analysis of the MD data using machine learning artificial intelligence, run on Kurnikova’s own computing cluster, helped tease these complex states apart. Also, water flow through the channel — an initial stand-in for ion current — increased in steps from one to three glutamates. The four-glutamate-bound channel didn’t have higher water flow, but the team suspected that their simulations weren’t quite capturing its behavior.
To nail down the actual current, though, they needed to perform massive computations. The ions move through the channel in a two-steps-forward, one-back way. So the data would be incredibly noisy. The scientists needed Bridges-2’s power as well as the machine-learning step to pick out that weak signal.
The Anton, Bridges-2, and cluster work all pointed in the same direction. Each kink opened the channel a little more to ion current, at least up to the point at which three of the four subunits had bound to glutamate. The collaborators reported their tour de force results in a paper in the prestigious journal Nature in May, 2022.
The scientists will need to carry out further work to clarify the activity of the four-glutamate-bound iGluR. But for now, they believe they have unraveled how this important but complicated protein works. It’s the first step in designing new drugs to affect the receptor, giving doctors new options for helping people with problems in this life-critical protein.